Abstract
With increasing use of nuclear energy for various purposes all over the world, there is a growing debate over
its ill-effects. Biological effects of radiation are dependent on the dose of radiation to which a person is
exposed, dose rate of exposure, distance from radiation source, shielding and type of radiation and also type
of cells and tissues being exposed with the most critically affected tissues in adults being the spermatocytes
in the testis, haematopoietic precursor cells in the bone marrow and crypt cells in the intestines.
Radiation injury can manifest early after the exposure, known as Acute Radiation Syndrome (ARS) or after a
latent period of many months to years. Classic clinical syndromes associated with ARS include the
Haematopoietic, Gastrointestinal, and Cerebrovascular (formerly known as cardiovascular and central
nervous) syndromes with local radiation injury presenting as Cutaneous Syndrome (CS). Four phases may be
identified in ARS- Prodromal Phase, Latent Phase, Stage of Manifest Illness and Death or Recovery Phase. Long
term effects of exposure include cancers, genetic damage and cataracts.
As controlled experiments regarding radiation exposure are neither feasible nor ethical, sources of
information are the various disasters as the atomic bomb detonations in Japan (in the second world war),
Chernobyl reactor explosion, Fukushima disaster (post-tsunami & following earthquake) in Japan and
Mayapuri (Delhi, India) cobalt leak being some of them.
Key words: Radiation injuries; health hazards; nuclear energy; radiation protection.
|
Introduction
With increasing use of nuclear energy for generating
power, therapeutic medical purposes and also for
nuclear terrorism, there has been also an increase in
radiation accidents and hence there is need for
adequate medical knowledge and response. The
nuclear debate is growing in importance as
governments everywhere are looking for ways to
maintain economic growth and reduce the effects of
global warming. But it now seems like the
disadvantages are just too great. Nuclear power
provides about 6% of the world's energy and 13–14%
of the world's electricity, with the U.S., France and
Japan together accounting for about 50% of nuclear
generated electricity [1,2]. Nuclear power is
controversial and there is an ongoing debate about
the use of nuclear energy. Proponents, such as the
World Nuclear Association and International Atomic
Energy Agency (IAEA), contend that nuclear power is
a sustainable energy source that reduces carbon
emissions. Opponents, such as Greenpeace
International and NIRS, believe that nuclear power
poses many threats to people and the environment
[3,4]. These threats include the problems of
processing, transport and storage of radioactive
nuclear waste, the risk of nuclear weapons
proliferation and terrorism, as well as health risks
and environmental damage from uranium mining.
They also contend that reactors themselves are
enormously complex machines where many things
can and do go wrong, and there have been serious
nuclear accidents [5,6]
Determinants of radiation injury- The amount of
radiation (i.e. radiation dose) absorbed by the
patient's tissues is highly predictive of its biological
effects. Such doses are defined as the amount of energy of ionising radiation deposited per unit of
tissue mass at a specific point. Some amount of
exposure naturally occurs during certain medical
imaging such as: a standard chest x-ray delivers a
dose of 6 to 11 mrem (0.06 to 0.11 mSv, 0.06 to 0.11
mGy). Interventional cardiologists working in a highvolume
catheterisation laboratory may have collar
badge exposures exceeding 600 mrem (6 mSv) per
year. A barium enema with 10 spot images delivers a
dose of approximately 0.7 rem (700 mrem, 7 mSv, 7
mGy). Similar doses (7-8 mSv) are delivered from a
CT scan of the chest or a PET scan, while a combined
PET/CT scan is estimated to deliver a dose of 25 mSv
[7,8,9]. But these exposures are not hazardous. The
lowest radiation dose resulting in an observable
effect in man on bone marrow depression, with a
resultant decrease in blood cell counts, is in the
range of 10-50 rem (100 to 500 mSv, 0.1 to 0.5 Gy).
The lowest total body dose at which the first deaths
may be seen following exposure to ionising radiation
is in the range of 1.0 to 2.0 Gy. Depending upon the
type of support given, 50 percent of people exposed
to a dose of 3 to 4 Gy will be expected to die of
radiation-induced injury. There is virtually no
chance of survival following a total body exposure in
excess of 10 to 12 Gy.The term “Lethal dose of
radiation” has thus been defined — which is the dose
associated with death in 50 percent of those
similarly exposed (i.e., the LD 50). Depending on the
incident, estimates for the LD 50 have ranged from
1.4 Gy among atomic bomb survivors in Japan to 4.5
Gy following uniform total-body exposure to
external photons [10,11].
Several factors determine the lethality of ionising
radiation. These include: ‘Dose rate’ (doses
received over a shorter period of time cause more
damage), ‘Distance from the source’ (For point
sources of radiation, the dose rate decreases as the
square of the distance from the source {inverse
square law}), ‘Shielding’ (Shielding can reduce
exposure, depending upon the type of radiation and
the material used, ‘Type of radiation’ (alpha
particles can be stopped by a sheet of paper or a
layer of skin, beta particles by a layer of clothing or
less than one inch of a substance such as plastic, and
gamma rays by inches to feet of concrete or less
than one inch of lead) [12].
Radiation exposure causes different degree of
damage to various body structures depending upon
certain inherent properties of cells. Radiosensitivity
varies directly with the ‘rate of cellular proliferation’. Rapidly dividing cells are more
profoundly affected. Radiosensitivity varies directly
with the ‘number of future divisions’. Long-lived
gonadal and haematopoietic stem cells fall into this
category. Radiosensitivity varies indirectly with the‘degree of morphologic and functionl
differentiation’. As an example, cells at the growth
plate in bone, which have not yet developed into
bone or cartilage, are more sensitive than those of
the diaphysis. Accordingly, growth arrest of bone is
commonly seen after radiation exposure to the
growth plate in children, as may occur in the
treatment of malignancy. Variation in sensitivity to
radiation is an inherited genetic trait, although
candidate gene studies have been largely
unsuccessful in identifying the genetic variants
underlying most phenotypes [13,14]. While all
tissues composed of short-lived cells are directly
and indirectly affected by radiation, the most
critically affected tissues in adults include the
following: spermatocytes in the testis,
haematopoietic precursor cells in the bone marrow
and crypt cells in the intestines.
Dose-dependent effects on various organs have also
been identified. They are of two types,
deterministic and stochastic: A ‘deterministic’
effect is one in which the severity is determined by
the dose (e.g. depression of blood counts). A dose
threshold (i.e. a dose below which an effect is not
seen) is characteristic of this effect. As an example,
the threshold absorbed dose for a "deterministic
effect" on bone marrow (0.5 Gy) is lower than that for all other organs, except for the testis (0.15 Gy). A‘stochastic’ effect represents an outcome for which
the probability of occurrence (rather than severity)
is determined by the dose. An example is radiationinduced
carcinogenesis, which occurs after a
prolonged and variable delay (latency) after
exposure. These effects do not have an apparent
threshold dose. The mechanisms underlying deterministic and stochastic effects remain
unknown. Studies showing the impact of radiation
on gene function may shed light in this area.
Radiation injury
The damage caused by radiation exposure can be
categorised according to whether the symptoms and signs develop immediately or are delayed by months
or years. The ensuing damage results from the
sensitivity of cells to radiation, with the most
rapidly dividing cells being the most sensitive to the acute effects of radiation. The inherent sensitivity
of these cells results in a constellation of clinical
syndromes that occur within a predictable range of
doses after a whole-body or significant partial-body
exposure. Symptoms arising from such exposures
are referred to as radiation sickness or acute
radiation syndrome (ARS). Classically, the threshold
dose for ARS is a whole-body or significant partialbody
irradiation of greater than 1 Gy delivered at a
relatively high dose rate.
Acute radiation syndrome
Acute changes, which are seen within the first two
months following exposure, include signs and
symptoms resulting mainly from damage to the skin,
central nervous system, lung, gastrointestinal tract,
and haematopoietic tissues. Classic clinical
syndromes associated with ARS include the
haematopoietic, gastrointestinal, and
cerebrovascular (formerly known as cardiovascular
and central nervous) syndromes, although there is
significant clinical overlap.
Local radiation injury, sometimes called the
Cutaneous Syndrome (CS), is especially common and
important in patients with ARS consequent to a nonuniform
exposure. The CS may include changes
ranging from epilation to radionecrosis. The
presence of ARS complicates the management of CS,
due to poor wound healing, infections and bleeding,
while the converse is also true. As an example,
severe CS dramatically affected the course of victims of the Chernobyl accident, and was the main
cause of death in more than half of lethal cases [15].
There are four main phases to the ARS [16]-
The Prodromal Phase usually occurs in the first 48
hours following exposure, but may develop up to six
days after exposure.
The Latent Phase is a short period characterised by
improvement of symptoms. However, this effect is
transient, lasting for several days to a month. The
duration of this phase is inversely related to the
dose of radiation received, and may be absent at the
highest, fatal doses.
The Stage of Manifest Illness may last for weeks, and
is characterized by intense immunosuppression. It is
the most difficult to manage. If the person survives
this stage, recovery is likely.
Death or Recovery Phase — Those patients who
recover will require close follow-up for the first
year, owing to the risk for unusual infections, as
aberrant immune reconstitution is probable in those
with significant exposure. Survivors will require lifelong
follow-up to monitor for long-term
complications, such as organ dysfunction and
carcinogenesis.
The onset, duration, and dominant pattern of the
acute radiation syndrome depend upon the dosage
of radiation received (Table 1) [17,18]. As examples,
the prodromal syndrome is often minimal in those exposed to doses of ≤ 1 Gy, while those exposed to
doses of 10 to 20 Gy may have a rapid compression of
phases and proceed from the prodromal phase to
death in two days or less.
Table 1- Phases of radiation injury
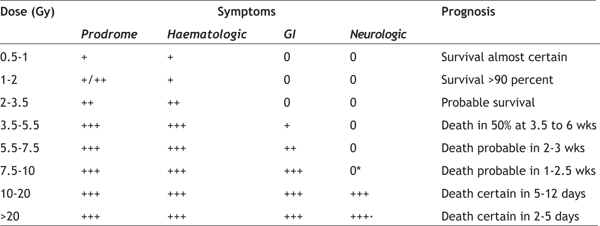
Gy: dose in Grey; GI: gastrointestinal effects; 0: no effects; +: mild; ++: moderate; +++: severe or marked.
* Hypotension. Also cardiovascular collapse, fever, shock.
The Prodromal Syndrome is generally mild or absent
at total body doses of 1 Gy or less. Patients whose
symptoms begin more than two hours after exposure
were probably exposed to doses <2 Gy. They can be
expected to fully recover within one month,
although long-term sequelae may develop. Onset of
symptoms within the first two hours usually
indicates significant and potentially lethal
exposures exceeding 2 Gy. At these doses, sloughing
of the gastrointestinal epithelium also occurs (i.e.
the gastrointestinal syndrome), adding to the
symptomatology. At doses between 2 to 10 Gy, it is
difficult to establish a prognosis based solely on the
existence and/or severity of the prodromal
syndrome. At high doses (e.g, 10 to >20 Gy),
prodromal symptoms occur in virtually all patients
within minutes of exposure [19,20,21]. These gradually
merge into loss of consciousness and hypotension,
components of the cerebrovascular syndrome.
Death often occurs within a few days to weeks after
such exposures. Accordingly, a rapid and severe
prodromal response is the harbinger of a poor
clinical outcome.
The Cerebrovascular Syndrome, also called the
neurovascular syndrome or CNS syndrome, results
from localised changes in the central nervous
system. These include impaired capillary circulation
with damage to the blood-brain barrier, interstitial
oedema, acute inflammation, petechial
haemorrhages, inflammation of the meninges, and
hypertrophy of perivascular astrocytes. Paroxysmal
spike and wave discharges may be evident on the
EEG, and the presence of swelling and oedema may
be documented by CT scan and MRI of head [22].
There may be a latent period of a few hours in which
there is apparent improvement, but within five to six hours watery diarrhoea, secondary to severe
gastrointestinal syndrome, respiratory distress,
fever, and cardiovascular collapse ensue. The final
picture, which may mimic that of sepsis, includes
hypotension, cerebral oedema, increased
intracranial pressure, and cerebral anoxia, with
death in about two days time.
The Gastrointestinal Syndrome typically develops
within five days of the initial exposure (Table 2)
[18]. At doses <1.5 Gy, only the prodromal phase of
nausea, vomiting, and gastric atony are observed
[23]. More severe symptoms develop at doses
between 5 and 12 Gy, secondary to loss of intestinal crypt cells and breakdown of the mucosal barrier,
with sloughing of the epithelial cell layer and
denudation of the bowel wall [24]. These changes
result in crampy abdominal pain, diarrhoea, nausea
and vomiting, gastrointestinal bleeding with
resultant anaemia, and abnormalities of fluid and
electrolyte balance. This early phase is often
followed by a latent phase lasting five to seven days,
during which symptoms abate. Vomiting and severe diarrhoea accompanied by high fever make up the
manifest illness. Systemic effects at this time may
include malnutrition from malabsorption.
Table 2- Radiation toxicity- gastrointestinal system

Impaired barrier function of the gastrointestinal
tract results in the passage of bacteria and their
toxins through the intestinal wall into the
bloodstream, predisposing to infection and sepsis,
which may be further compromised by
immunosuppression and cytopenias (secondary to
development of the haematopoietic syndrome).
Other severe complications include ulceration and
necrosis of the bowel wall, leading to stenosis,
ileus, and perforation. In the latter case, recovery is
most unlikely, as radiosensitive stem cells in the
crypts of the gastrointestinal tract are permanently
damaged. Consequently, there is no replacement of
cells that are lost from the surface of the villi
through the sloughing process, precluding recovery [25]. However, mild gastrointestinal symptoms
limited to one or two episodes of diarrhoea with
associated abdominal pain are accompanied by
virtually certain recovery, provided that the
haematopoietic syndrome which follows is
reversible.
The Haematopoietic Syndrome develops at doses
exceeding 1 Gy and is rarely clinically significant at
doses <1 Gy [20,21,24,26,27]. Mitotically active
haematopoietic precursors have limited capacity to
divide after whole-body doses greater than 2 - 3 Gy. Neutropenia and thrombocytopenia reach a nadir at
two to four weeks and may persist for months.
Anaemia inevitably ensues, due to the combined
effects of gastrointestinal blood loss from the
gastrointestinal syndrome, haemorrhage into
organs and tissues secondary to thrombocytopenia,
and ultimately, bone marrow aplasia. In the ensuing
weeks to months after exposure, hypoplasia or
aplasia of the bone marrow occurs, resulting in
pancytopenia, predisposition to infection,
bleeding, and poor wound healing, all of which may
contribute to death in the absence of appropriate
supportive care. Lymphopenia is common and
occurs before depression of other cellular elements,
and may develop within the first 6 to 24 hours after
exposure to a moderate or high dose [25,28,7].
Based on the overall levels of lymphocyte,
neutrophil, and platelet counts, as well as the
presence or absence of infection and blood loss, the
relative severity of toxicity to the haematopoietic
system can be evaluated (Table 3) [18].
Table 3- Levels of haematopoietic toxicity following radiation exposure
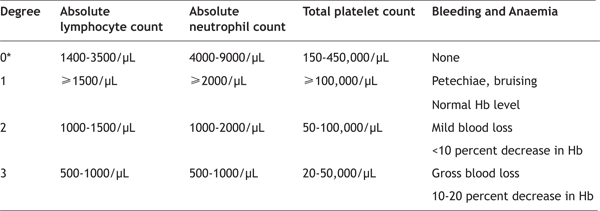
* Degree 0 represents normal reference values.
The Cutaneous Syndrome may develop early following exposure (e.g. one to two days). However,
it may take years before becoming fully manifest.
Early lesions include erythema, oedema, and dry
desquamation of the skin. Such lesions may be
isolated or may appear simultaneously in several
locations, depending on the amount of skin
receiving direct exposure. More advanced lesions
include bullae, moist desquamation, ulceration,
and onycholysis. The severity of the cutaneous
reaction depends upon the depth dose distribution
of the radiation source.
- Blisters and bullae with or without necrosis
appear one to three weeks after localised
exposure to doses of >30 Gy [29,30].
- Moist desquamation and ulceration are seen
with localised doses of 20 to 25 Gy [30].
- The estimated threshold for erythema is a
localised exposure dose of 10 to 15 Gy.
- Epilation occurs 10 to 20 days after a single
localised exposure to 3 to 4 Gy or greater.
Delayed effects
Long Term Radiation Exposure results from residing
in a fallout contaminated area for an extended
period (external exposure), consuming food
produced in a contaminated area (internal
exposure), or both. If the exposure rate is low
enough, no symptoms of radiation sickness will
appear even though a very large total radiation dose
may be absorbed over time. Latent radiation effects
(i.e. cancer, genetic damage) depend on total
dosage, not dose rate, so serious effects can result.
Internal Exposure: Radioisotopes may be taken up into plants through the root system, or they may be
contaminated by fallout descending on the leaves.
The primary risks for internal exposure are caesium-137 and strontium-90. Strontium-89, transuranics
alpha emitters, and carbon-14 are also significant
sources of concern. Caesium-137 is readily absorbed
by food plants, and by animal tissues and distributes
itself fairly evenly through the body causing whole
body exposure. Strontium is chemically similar to
calcium, and is deposited in bone along with
calcium. Somewhat less than 10% of the strontium is
retained in the bone, but since bone marrow is
among the most sensitive tissue in the body to
radiation, this creates a very serious hazard. If small
particles of alpha emitters are inhaled, they can
take up permanent residence in the lung and form a
serious source of radiation exposure to the lung
tissue. Uranium and the transuranic elements are
bone-seekers (with the exception of neptunium)
and present a serious exposure risk to bone tissue
and marrow.
Cancer: The most serious long term consequence of
radiation exposure is the elevation of cancer risk.
Cancer risk is more or less proportional to total
radiation exposure, regardless of the quantity, rate
or duration. There is no evidence of a "safe dose".
Safety standards are established primarily to keep
the increased incidence of cancer below detectable
levels. Cancer risk to radiation exposure can be
expressed as the increase in the lifetime probability
of contracting fatal cancer per unit of radiation. The
current estimate of overall risk is about a 0.8%
chance of cancer per 10 rem for both men and
women, averaged over the age distribution of the
U.S. population. There is also risk coefficient for
specific tissue exposures (approximately):
Female Breast
Bone Marrow
Bone Tissue
Lung |
1.0%/100 rem
0.2%/100 rem (0.4% for children)
0.05%/100 rem
0.2%/100 rem |
Genetic Effects: Radiation damage to germ cells of
the reproductive organs can cause mutations that
are passed on to subsequent generations. However,
no elevated mutation rate from radiation has ever
been detected even in the substantial population of
atomic bomb survivors and descendants. Two
factors can explain this- High acute exposures to the
reproductive organs can cause permanent sterility,
which prevents transmission of genetic effects and
cumulative effect of chronic exposure is limited by the fact that only exposures prior to reproduction
count. Since most reproduction occurs before the
age of 30, exposures after that age have little effect
on the population.
Cataracts: Eye tissues exposed to radiation show an
increased incidence of cataracts at dose levels
below which most tissues show increased cancer
rates. This makes cataract risk the most important
tissue dose criterion for establishing safety
standards [31].
Source of information
Our understanding of the effects of total-body
radiation is derived from analysis of the clinical
course of individuals exposed to radiation after the
detonation of two atomic bombs over Japan in 1945,
as well as radiation accidents that have occurred
throughout the world since that time. In some cases,
this includes a large affected population (e.g. the
Marshallese exposed in 1954 and individuals in the
former Soviet Union and Europe exposed during the
Chernobyl nuclear power plant disaster in 1986). As
examples: the Chernobyl reactor explosion in the
former Soviet Union resulted in high levels of
exposure, with 28 people receiving doses >6 Gy, 23
receiving 4 to 6 Gy, and 53 receiving 2 to 4 Gy [32].
There were 115 cases of acute radiation syndrome
and 28 deaths. In 1987, in Goiania, Brazil, an
abandoned Caesium-137 teletherapy source was
breached, with hundreds of people exposed to
gamma and beta radiation [33]. There were 48
hospitalisations for radiation injury and four deaths.
In contrast, the reactor breach at Three Mile Island
in the United States was calculated to result in no
more than 50 to 70 mrem of additional exposure to
any individual within range. In other cases,
relatively low numbers of individuals have been
exposed.
The Chernobyl nuclear power plant led to
radioactive contamination of aquatic systems which
became a major problem in the immediate
aftermath of the accident [34]. In the most affected
areas of Ukraine, levels of radioactivity
(particularly radioiodine: I-131, radiocaesium: Cs-
137 and radiostrontium: Sr-90) in drinking water caused concern during the weeks and months after
the accident. Bio-accumulation of radioactivity in
fish was seen to be significantly above guideline
maximum levels for consumption [34]. Groundwater was not badly affected by the Chernobyl accident
since radionuclides with short half-lives decayed
away long before they could affect groundwater
supplies, and longer-lived radionuclides such as
radiocaesium and radiostrontium were adsorbed to
surface soils before they could transfer to
groundwater [35]. However, significant transfers of
radionuclides to groundwater have occurred from
waste disposal sites in the 30 km exclusion zone
around Chernobyl. After the disaster, four square
kilometres of pine forest directly downwind of the
reactor turned reddish-brown and died, earning the
name of the "Red Forest". Some animals in the
worst-hit areas also died or stopped reproducing. Up
to the year 2005, more than 6,000 cases of thyroid
cancer have been reported in children and
adolescents who were exposed at the time of the
accident. There is no scientific evidence of increase
in overall cancer incidence or mortality rates or in
rates of non-malignant disorders that could be
related to radiation exposure [36].
In April 2010, a 35 year old man died after exposure
to scrap metal containing Cobalt-60 in the Mayapuri
industrial area of New Delhi [37]. The man died of
multiple organ failure when various treatment
modalities failed to resuscitate him. Six other
people from the same area were also hospitalised
after being exposed to the contaminated scrap
metal. Officials retrieved 11 samples of
contaminated materials containing Cobalt-60,
which is a radioactive material used in food
irradiation and radiotherapy.
Following an earthquake, tsunami, and failure of
cooling systems at Fukushima I Nuclear Power Plant
and issues concerning other nuclear facilities in
Japan on March 11, 2011, a nuclear emergency was
declared with explosions and a fire resulting in
dangerous levels of radiation. This was the first time
a nuclear emergency had been declared in Japan,
and 140,000 residents within 20km of the plant were
evacuated [38].
Key Points
- Harmful effects of radiation have been
evident, during nuclear accidents/disasters.
- Acute and delayed damage can occur following
radiation exposure.
- Various factors, determine the extent and
pattern of injury including the nature and dose
of radiation and tissue characteristics.
- Hence, nuclear energy harnessing has to be
viewed in light of all the hazards it poses to the
human beings and their environment.
|
References
- World Nuclear Association. Another drop in
nuclear generation World Nuclear News, 05
May 2010.
- (PDF) Key World Energy Statistics 2007.
International Energy Agency. 2007.
http://www.iea.org/textbase/nppdf/free/20
07/key_stats_2007.pdf. Retrieved 2008-06-21.
- Share. "Nuclear Waste Pools in North Carolina".
http://www. projectcensored.org/topstories/articles/4-nuclear-waste-pools-innorth-
carolina/. Retrieved 2010-08-24.
- Sturgis S. "Investigation: Revelations about
Three Mile Island disaster raise doubts over
nuclear plant safety". Southernstudies.org.
http://www.southernstudies.org/2009/04/post-4.html. Retrieved 2010-08-24.
- Sovacool BK. The costs of failure: A preliminary
assessment of major energy accidents, 1907–2007. Energy Policy 36, 2008, pp. 1802-20.
- Cooke S. In Mortal Hands: A Cautionary History
of the Nuclear Age, Black Inc. 2009, p. 280.
- Rotblat J. Acute mortality in nuclear war. In:
The Medical Implications of Nuclear War,
Soloman F, Marston RQ (Eds), Institute of
Medicine, National Academy of Sciences,
Washington, DC 1986,251-81.
- Mole RH. The LD50 for uniform low LET
irradiation of man. Br J Radiol 1984; 57:355-69.
- Reeves GI. Radiation injuries. Crit Care Clin
1999; 15:457-73.
- Bentzen SM. Preventing or reducing late side
effects of radiation therapy: radiobiology
meets molecular pathology. Nat Rev Cancer
2006; 6:702-13.
- Barnett GC, West CM, Dunning AM, Elliott RM,
Coles CE, Pharoah PD, et al. Normal tissue
reactions to radiotherapy: towards tailoring
treatment dose by genotype. Nat Rev Cancer
2009; 9:134-42.
- Amundson SA, Bittner M, Meltzer P, Trent J,
Fornace AJ Jr. Physiological function as regulation of large transcriptional programs:
the cellular response to genotoxic stress. Comp
Biochem Physiol B Biochem Mol Biol 2001;
129:703-10.
- Amundson SA, Do, KT, Shahab S,
Meltzer P, Trent J, et al. Identification of
potential mRNA biomarkers in peripheral blood
lymphocytes for human exposure to ionising
radiation. Radiat Res 2000; 154:342-6.
- Goldberg Z, Schwietert CW, Lehnert B, Stern R,
Nami I. Effects of low-dose ionising radiation
on gene expression in human skin biopsies. Int J
Radiat Oncol Biol Phys 2004; 58:567-74.
- Lushbaugh CC. Human radiation tolerance. In:
Parker J Jr (Ed), Bioastronautics Data Book.
NASA-S-30006. National Aeronotics and Space
Administration Washington, DC 1973; 421.
- Wald N. Radiation Injury. In: Wyngaarden JB,
Smith LH Jr (Eds). Cecil Textbook of Medicine,
WB Saunders, Philadelphia 1982, p.2228.
- Anno GH, Baum SJ, Withers HR, Young RW.
Symptomatology of acute radiation effects in
humans after exposure to doses of 0.5-30 Gy.
Health Phys 1989; 56:821-38.
- Waselenko JK, MacVittie TJ, Blakely WF, Pesik
N, Wiley AL, Dickerson WE, et al. Medical
management of the acute radiation syndrome:
Recommendations of the strategic national
stockpile radiation working group. Ann Int Med
2004; 140:1037-51.
- Fliedner TM, Friesecke I, Beyrer, K. Medical
Management of Radiation Accidents: Manual on
the Acute Radiation Syndrome. British Inst
Radiol, Oxford 2001;27-32.
- Gangloff H. Hippocampal spike activity
following low doses of irradiation. In: Response
of the Nervous System to Ionising Radiation,
Haley TJ, Snider RS (Eds), Little Brown, Boston
1964:221-42.
- Dubois A, Walker RI. Prospects for management
of gastrointestinal injury associated with the
acute radiation syndrome. Gastroenterology
1988; 95:500-7.
- Goans RE, Holloway EC, Berger ME, Ricks RC.
Early dose assessment in criticality accidents.
Health Phys 2001; 81:446-9.
- Husebye E, Hauer-Jensen M, Kjorstad K, Skar V.
Severe late radiation enteropathy is
characterized by impaired motility of the
proximal small intestine. Dig Dis Sci 1994;
39:2341-49.
- Medical Consequences of Nuclear Warfare.
Walker RI, Cerveny RJ (Eds) Office of the
Surgeon General, Falls Church, VA 1989.
Available at www.afrri.usuhs.mil (Accessed
8/10/04).
- Hall EJ. Radiobiology for the Radiologist, 5th
ed, Lippincott, Williams & Wilkins,
Philadelphia 2000;91-111.
- Andrews GA, Cloutier RJ. Accidental acute
radiation injury. The need for recognition. Arch
Environ Health 1965; 10:498-507.
- Rezvani M, Hopewell JW, Wilkinson JH, Bray S,
Morris GM, Charles MW. Time- and dose-related
changes in the thickness of skin in the pig after
irradiation with single doses of thulium-170
beta particles. Radiat Res 2000; 153:104-9.
- Peter RU. Cutaneous radiation syndrome -
clinical and therapeutic aspects. Rad Protect
Bull 1996; 183:19-25.
- Gale RP. Immediate medical consequences of nuclear accidents. Lessons from Chernobyl.
JAMA 1987; 258:625-28.
- Roberts L. Radiation accident grips Goiania.
Science 1987; 238:1028-31.
- Gerusky TM. Three Mile Island: assessment of
radiation exposures and environmental
contamination. Ann N Y Acad Sci 1981; 365:54-9.
- Ricks RC. The radiation-accident patient in the
new millennium: past history and future
threats. In: The Medical Bases for Radiation-
Accident Preparedness: The Clinical Care of
Victims, Ricks RC, Berger ME, O'Hara FM (Eds),
The Parthenon Publishing Group, Boca Raton,
FL 2002. p.1.
- Dainiak N, Ricks RC. The evolving role of
haematopoietic cell transplantation in
radiation injury: potentials and limitations.
BJR Suppl 2005; 27:169-74.
- Wildlife defies Chernobyl radiation, by Stefen
Mulvey, BBC News. news.bbc.co.uk/2/hi/europe/4923342.stm Published April 20,
2006. (Retrieved 03/05/2011).
- http://en.wikipedia.org/wiki/List_of_civilian_radiation_accidents#2010
(Retrieved 03/05/2011)
- Clarens F; de Pablo J, Diez-Perez I, Casas I,
Gimenez J, Rovira M (2004-12-01). "Formation
of Studtite during the Oxidative Dissolution of
UO2 by Hydrogen Peroxide: A SFM Study".
Environmental Science & Technology 38 (24):
6656–6661.
- Indian Man Dies after Radiation Exposure. http://www.nytimes.com/2010/04/28/world
/asia/28india.html (Retrieved 03/05/2011).
- Weisenthal Joe (11 March 2011). "Japan
Declares Nuclear Emergency, As Cooling
System Fails At Power Plant". Business Insider.
http://www.businessinsider.com/fukushimanuclear-plant-2011-3.(Retrieved 03/05/2011)